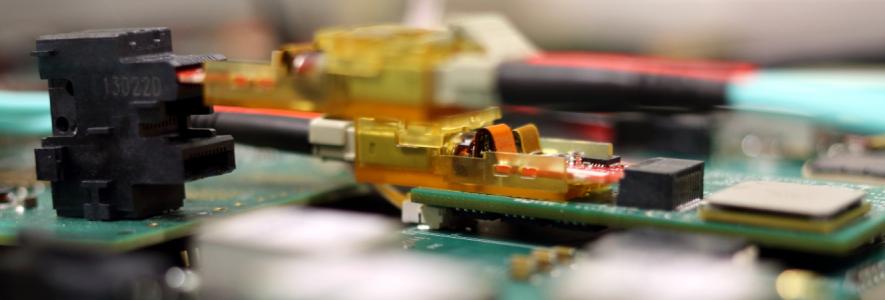
LHCb RICH Upgrade
The Cambridge group is responsible for the design of electronics hardware and firmware for the upgrade of the LHCb RICH (Ring-imaging Cherenkov) detectors. RICH detectors are able to identify the type of particles that pass through them by detecting the Cherenkov radiation emitted by the particles as they exceed the local speed of light inside the detector.
Cherenkov Radiation
Cherenkov radiation is produced when a charged particle travels faster than the local speed of light in a ‘dielectric’ material
In space, light travels at 300,000 kilometers a second; a fundamental speed limit that cannot be exceeded. That means that a photon can cover a distance equivalent to seven-and-a-half laps of the earth’s equator every second. However, when light passes through a material, it can travel a lot slower. For example, in glass, light travels at about 2/3 of the speed it does in air.
A particle accelerator, such as the LHC, is capable of accelerating particles so that they travel faster than the local speed of light in any material that is in their way. When this happens to a charged particle and the material it passes through has certain electrical properties, it creates an electromagnetic shockwave – analogous to the sonic boom that happens when an object travels faster than the speed of sound.
This shockwave appears as blue or ultraviolet photons that are emitted at a very specific angle from the direction of travel of the original particle. This angle is dependent only on the speed of the particle and the properties of the material it was passing through, which makes it useful for identifying particles.
The characteristic purple-blue colour of Cherenkov radiation is also the cause of the ghostly blue glow seen in pictures of nuclear reactors.
More information on Cherenkov Radiation can be found here and here.
RICH detector
The RICH detector uses the unique properties of Cherenkov light to identify particles by their momentum
In the LHCb RICH detectors, the LHC beam passes through a specific material, known as a ‘radiator’, in which high energy charged particles emit Cherenkov light. In LHCb, the radiators for both RICH 1 and RICH 2 are gases, each optimised to detect different types of particles.
The Cherenkov light emitted in the radiator is first reflected off a flat mirror, before being reflected off a spherical mirror and on to a photon detector. The geometry of these mirrors is such that all the Cherenkov light from a particle forms a ring on the photon detector, where the diameter of the ring depends on the particle’s speed. Knowing the momentum of given particle, measured elsewhere in LHCb, its mass can be calculated, based on the size of the ring it leaves in the RICH.
To detect these rings, the photon detector must be sensitive to individual photons and capable of accurately measuring the size of the rings. In the LHCb RICH, four separate photon detector planes are used, with around 250,000 individual pixels between them. These are capable of imaging the Cherenkov rings every 25 ns. In effect, the RICH detectors are like a digital camera that can take 40 million images per second.
The RICH detector acts like a digital camera that can detect single photons and takes 40 million photographs per second
This creates a vast amount of data that must be transmitted from the detector to the surface for processing. This is dealt with by the digital boards, designed at Cambridge. These read out the entire detector and transmit the data over more than 3,500 fibre optic links at a combined rate of more than 17 Terabits per second. That’s about the same data rate you’d need to stream 320,000 high-definition videos at once!
The LHCb RICH upgrade
The two RICH detectors in LHCb are being upgraded over the next LHC long shutdown in 2018 and 2019. This upgrade will enable the experiment to capture events at 40 times today's rate at a greater luminosity than today, whilst also allowing more precise particle identification. This involves a complete re-design of the front-end electronics to allow them to take advantage of the most recent developments in photodetectors and electronics.
The architecture for both RICH 1 and RICH 2 is similar. Arrays of 'multi-anode photomultiplier tubes' (MaPMTs) are used to detect individual Cherenkov photons, with a resolution of a few mm. The signals from these MaPMTS are tiny - each photon causes an electrical signal consisting of a few hundred thousand electrons that lasts only a few billionths of a second.
The signals detected by the front-end electronics are pulses of a few hundred thousand electrons, each lasting just a few billionths of a second
To avoid these tiny signals being swamped by the electrical noise of the rest of the detector, they are immediately fed into the front-end 'CLARO' chips. These radiation-hard chips are custom-designed for the LHCb RICH and are responsible for amplifying and buffering the MaPMT signals, so that each time a photon hits the detector, a corresponding digital pulse is transmitted to the digital board.
The signals from up to 8 MaPMTs - 512 channels in all - are fed to the digital board, currently being developed by the Cambridge group. The digital board has two main functions. Using FPGAs, it firstly synchronises the data from the MaPMTs so that the signals to from each are correctly identified as having occuring during a particular set of collisions (known as a 'bunch crossing'). It then transmits the data as a high-speed serial stream of data over a series of optical fibre links to the control systems at the surface, where interesting events will be selected and retained for storage and later analysis.
The digital board is also responsible for the overall control of the front end of the experiment, such as configuring the CLARO chips, programming the FPGAs and monitoring the status of the experiment during operation.
As well as the design of the digital board, the Cambridge group is also involved with designing the power supply for the RICH, as well as optimising the grounding for the detector and investigating further improvements for future upgrades.