Professor Mark Thomson
Professor of Experimental Particle Physics
Professorial Fellow of Emmanuel College
Executive Chair of STFC
Group: High Energy Physics
Cambridge Address:
Room 951 Rutherford Building,
Cavendish Laboratory,
JJ Thomson Avenue,
Cambridge CB3 0HE.
Tel: +44 (0)1223 765122
Email: thomson "at" hep.phy.cam.ac.uk
Modern Particle Physics
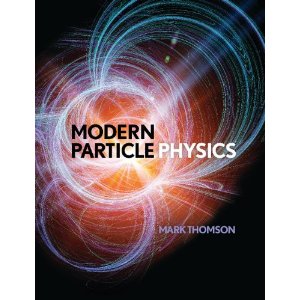
Modern Particle Physics is a widely-used up-to-date textbook aimed at final year undergraduate students and first year graduate students. It covers all topics ain contemporary particle physics and is accessible to students with a grounding in quantum mechanics and special relativity.
Modern Particle Physics is published by Cambridge University Press. Further details can be found on the CUP web pages.
Biographical Information
2018-              | Executive Chair of STFC |
2015-2018              | Co-spokesperson of the DUNE collaboration |
2008-              | Professor of Experimental Particle Physics at the Cavendish Laboratory and Professorial Fellow of Emmanuel College, Cambridge |
2004-2008 | Reader in Experimental Particle Physics at the Cavendish Laboratory and Fellow of Emmanuel College, Cambridge |
2000-2004 | University Lecturer in Physics at the Cavendish Laboratory and Fellow of Emmanuel College, Cambridge |
1996-2000 | Staff Research Physicist, CERN, Geneva, Switzerland |
1994-1996 | CERN Fellow, CERN, Geneva, Switzerland |
1992-1994 | Research Fellow in the High Energy Physics group, University College London, London. |
1988-1991 | D.Phil. in Experimental Particle Astrophysics in the Department of Nuclear Physics, Oxford |
1985-1988 | BA Physics at the University of Oxford |
Research Summary
My main research interests are neutrino physics, the physics of the electroweak interactions, and the design of detectors at a future colliders. I am co-spokesperson of the DUNE collaboration, which consists of over 1000 scientiests and engineers from over 170 institutions in 31 nations across the globe. The Cambridge neutrino group splits its acivities between MicroBooNE and DUNE and is using advanced particle flow calorimetry techniques to interpret the images from large liquid argon TPC neutrino detector.
DUNE
Overview
DUNE will be the largest particle physics project ever undertaken in the US. It involves firing an intense neutrino beam from Fermilab, near Chicago, to the DUNE far detector in the Sanford Underground Research Facility (SURF) in South Dakota; a distance of 1300 km. The DUNE far detector is conceived as four vast liquid argon time projection chambers (LAr-TPCs), each consisting of an active volume of 10,000 tons of liquid argon. The LAr-TPC technology provides 3D images of the particles produced in neutrino interactions. The experiment aims to discover CP violation in neutrino oscillations, which could explain the observed excess of matter over antimatter in the Universe. It also enables a powerful search for proton decay, which is predicted in Grand Unified theories, and is sensitive to neutrinos from core collapse super-novae. DUNE is a major international collaboration of over 1000 scientists and engineers from aroudn the globe. The installation of the first far detector is module is planned for 2022.
The UK in DUNE
Cambridge and DUNE
This work also formed the basis for the more recent developments in LAr-TPC reocnstruction. This was possible due to the
transformation of the intial particle flow software into a fully-fledged pattern recognition framework, the Pandora SDK.
As a result the Pandora-based reconstruction techniques are at the forefront of both the development of detectors future
electron-positron colliders and the LAr-TPC neutrino programme.
Professor Thomson played a central role in the design and optimisation of the detectors for the ILC and CLIC,
focussing on the ILD detector concept for high-granularity particle flow calorimetry. The development of the
pandoraPFA software provided, for the first time, a concrete demonstration of the power of particle flow
calorimetry in a high-energy electron-positron collider.
Professor Thomson also worked on the physics case for the ILC and, in particular CLIC, focusing on precision
measurements of the Higgs Boson couplings to other particles. In a relativelt recent publication he demonstrated
that the fully-hadronic Z Boson decays in ZH events could be used to make a model-independent measurement of
the cross section. This is signifcant because it argues against the need to operate a Linear Collider at
a centre-of-mass energy of 250 GeV in order to measure the invisible width.
Professor Thomson's main research activities with the OPAL experiment at CERN,
centred on the precision experimental measurements of
the properties of the W± and Z bosons responsible for
mediating the neutral and charged current weak interactions.
He performed a number of important measurments at both LEP 1 (e+e- -> Z0)
and LEP 2 (e+e- -> W+W-).
Including:the first
experimental limits on possible anomalous quartic gauge
boson couplings by studying hard photon radiation in
WW events;
measurments of the WW cross-section and, in particular,
the OPAL measurements of the
e+e- -> W+W- -> qqlvl
cross-section;
devoloped of algorithms to identify
e+e- -> W+W- -> qqlvl
events with high efficiency; leading the OPAL measurements of the
W boson mass at and above threshold; measurements of the tau polarization at LEP 1 using
the method of Maximum Entropy for the reconstruction of ECAL
clusters in the OPAL detector; measurements of the tau-pair production cross section as inputs to the global
electroweak fits; and measurements of the luminosity at LEP 1, which was another essential component to the
electroweak fits
Professor Thomson is the leader of the DUNE-UK project and secured STFC grants amounting to almost £7M support 16 UK institutions through the "development" and "pre-construction" phases (2015-2019). He was the scientific lead in the £65M UK investment in the DUNE construction project that was announced by the UK Science Minister in September 2017. The UK is playing a leading role in DUNE and will contribute to the construction of: the detector readout wire planes; the detector data acquisition system; SRF and cryo-modules for the PIP-II accelerator; and the LBNF neutrino target.
Cambridge has been a member of the predecesor LBNE collaboration since the very early days of the experiment concept.
In 2014, the collaboration was reformulated into the Long Baseline Neutrino Facility (LBNF) and the Deep Underground
Neutrino Experiment (DUNE), with increased international involvement.
We are currently working on the reconstruction of the neutrino interactions in the LBNF LAr TPC. The Cambridge DUNE group uses state-of-the-art
PandoraPFA reconstruction tools, initially
developed for particle flow reconstruction at a future linear collider. The reconstruction of LBNF events is particularly
challenging due to the higher energy neutrino beam spectrum that results on complex final states.
The MicroBooNE Experiment
Cambridge joined MicroBooNE in 2013.
The MicroBooNE detector is a large 170 ton Liquid
Argon (LAr) Time Projection Chamber (TPC) located along the Booster Neutrino Beam (BNB) line
The experiment will measure low energy neutrino cross sections and investigate the low energy
excess events observed by the MiniBooNE experiment. The detector serves as the necessary next
step in a phased program towards the construction of massive kiloton scale LArTPC detectors for
the LBNE experiment. The experiment has been operational since August 2017.
The Cambridge MicroBooNE group is working on state-of-the art reconstruction software for
extracting physics observables from the "photograph-like" images of neutrino interactions
in the LAr TPC. This work represents the first end-to-end fully-automated reconstruction of LAr-TPC
images and is a central to the anaysis of MicroBooNE data.
Particle Flow Calorimetry
Professor Thomson pioneered the technique of high-granularity Particle Flow Calorimetr, which
is a new technique for large-scale particle detectors at future colliders such as the ILC and CLIC.
He was the first to demonstrate definitavely that this technique can provide improvements of more than a factor of two
in jet energy resolution compared to conventional calorimetry. This development lies at the heart of the design of the detectors
for the ILC and CLIC.
The MINOS Experiment
The MINOS experiment is a second-generation Long Baseline (LBL) neutrino oscillation
experiment. A beam of muon neutrinos is produced
from protons extracted from the Main Injector at Fermilab (just outside
Chicago). These are then detected 735 km away in the main 5400 ton MINOS Far
Detector half a mile underground in the Soudan mine.
In addition a much smaller
(1000 ton) Near Detector, is located 290 m from the decay pipe. If
neutrinos have mass then some of the muon neutrinos will oscillate to
become tau neutrinos during the journey to the Soudan mine. Neutrino
oscillations are investigated by studying and comparing the rates and
energy spectra of Charged Current interactions in the MINOS near and
far detectors. Beam data taking commenced in March 2005 and ended in 2015. Professor Thomson and the Cambridge MINOS
group played a major role in the MINOS research activities including:
The ILC and CLIC
The ILC and CLIC are two possible options for future colliders after the LHC. Both are
electron-positron colliders and focus on precision measurements rather than direct probes of
the high-energy scale at hadron collider such as the LHC. The International Linear Collider (ILC)
would be a lower energy machine, most likely operating in the range 250 - 500 GeV. The
Compact Linear Collider (CLIC) is a higher-energy option (350 GeV - 3 TeV). The ILC is
currently under discussion for Japan. CLIC provides a possible future option for CERN.
The ATLAS Experiment at the LHC
The ATLAS experiment at the Large Hadron Collider (LHC) at CERN is one of the two large experiments
at the forefront of the energy frontier of particle physics. One of the main challenges is separating the interesting
interactions from the less interesting interactions. Because of the very high event rates the first stage on trigger on
on interesting events has to be done in real time in the 25ns between bunch crossings. From 2012-2015 Professor Thomson
managed the project to upgrade the first level hardware calorimeter trigger, where the
UK is developing a new system for the Phase-1 luminosity upgrade of the LHC.
The OPAL Experiment at LEP
The
OPAL
experiment ran from 1989-2000. It was designed to test the Standard Model
of the electroweak interaction with unprecedented precision.